Smart Radios for Smart Life
What smart impact will future 5G and IoT bring to our lives? Many may wonder, and even speculate, but do we really know? Our recent research focus is to unlock such a mystery by developing a new radio analytics framework to provide a foundation to decipher our world.
With more and more bandwidth readily available for the next generation of wireless applications, many more smart applications/services unimaginable today may be possible. With more bandwidth, one can see many multi-paths, which can serve as hundreds of virtual antennas/sensors that can be leveraged as new degrees of freedom for smart life applications.
Together with the fundamental physical principle of time reversal to focus energy to some specific positions, or more generally by employing waveforming, a revolutionary smart radio platform can be built to enable many cutting-edge IoT applications that have been envisioned for a long time, but have never been achieved. In a certain sense, we can create an Internet of Virtual Things by creating hundreds of virtual antennas/sensors on demand to suit our purposes.
WATCH
"Smart Radios for Smart Life", Plenary, IEEE International Conference on Acoustics, Speech, and Signal Processing (ICASSP), New Orleans, March 7, 2017.(Plenary Talk by Dr. Liu)
Time-Reversal Indoor Positioning with Centimeter-Accuracy
Recently, handheld and portable devices have become an important and indispensable part of our daily lives. To successfully and seamlessly provide users with services through these digital portals they carry around, the exact locations of the users are crucial for the service providers. However, locating users indoor is a very challenging task because the transmitted signals are distorted by the numerous multipaths created by the rich-scattering indoor environment.
In SIG lab, we solve the indoor positioning problem by utilizing the time reversal (TR) technology and turning the multipath into the crucial element for precise indoor positioning. By using large bandwidth, TR technology observes the location-specific multipath profiles in a rich scattering indoor environment. TR use the location-specific multipath profile to create the unique spatial focusing effect that focuses the transmitted energy precisely at the intended location.
SIG lab develops the TR indoor positioning system (TRIPS) prototype using the unique spatial focusing effect of the TR technology. TRIPS is the first system to perform centimeter level accuracy in indoor positioning with only one pair of antennas in the non-line-of-sight setting. Such astonishing precision makes possible a lot of location-dependent services, and bears a lot of potential for a broad range of applications.
See DemoCentimeter-Accuracy Indoor Positioning with WiFi
With time evolves, indoor localization is becoming increasingly more important, since people spend more time indoor nowadays. Conventional localization techniques, such as the well-known and widely used Global Positioning System (GPS), would be a non-starter for indoor localization, mainly because the severe signal attenuation and multipath propagation.
Realizing the shortcomings of GPS, many indoor localization systems (IPSs) have been proposed recently. Some of them utilize triangularization by deploying anchors into indoor environment and measure the anchor-to-device distances via some distance-relevant measures, for instance, received signal strength indicator (RSSI), known as the ranging methods, while for the others, location-specific fingerprints are collected in the offline phase and compared against instantaneous fingerprints in the online phase, known as the fingerprint methods. However, their accuracies degrade significantly in the presence of a large number of multipaths components as well as a lack of sufficient bandwidth to resolve enough independent multipaths components, which is common for indoor environment. Such limitations impose constraints on the achievable localization accuracy.
In this research, we show that centimeter-level accuracy is feasible for indoor localization using commercial WiFi devices with only limited bandwidth and with strong non-line-of-sight (NLOS) conditions. Instead of RSSI, we leverage channel state information (CSI), a fine-grained information describing the electromagnetic wave propagation. In the offline phase, we collect CSIs from multiple locations-of-interest from several WiFi bands and/or several antenna links, which are stored in a database. Then, in the online phase, we obtain instantaneous CSIs. The similarities between the instantaneous CSIs and the CSIs in the database are calculated using the metric of time-reversal resonating strength (TRRS). In the calculation of TRRS, we concatenate the available bandwidths from multiple WiFi bands and/or antenna links, achieving a large effective bandwidth. Moreover, the phase distortion embedded in the CSIs are corrected in the calculation of TRRS. Then, by comparing TRRS values with a predefined threshold, we can match the device location uniquely to one of the locations measured in the offline phase. We perform extensive experiments in different indoor environment to verify that a large effective bandwidth leads to high accuracy indoor localization
See DemoInfrastructure-free Indoor Tracking with Sub-meter Accuracy
Although outdoor localization has been successfully completed by GPS, indoor localization still leaves an open problem. The indoor RTLS market is estimated to reach 4 billion by 2020. More than ever before, enterprises of all sizes are investing in RTLS technology to support a growing list of applications. Such RTLS applications include patient tracking, asset management, workflow automation, and safety alerting. Recently, new applications, such as Facebook Nearby, Yelp, and Shopkick, trigger the research community to introduce commodity smartphones for indoor localization. The key demands of RTLS can be summarized as high accuracy, real time, low cost, energy efficiency and privacy.
See Demo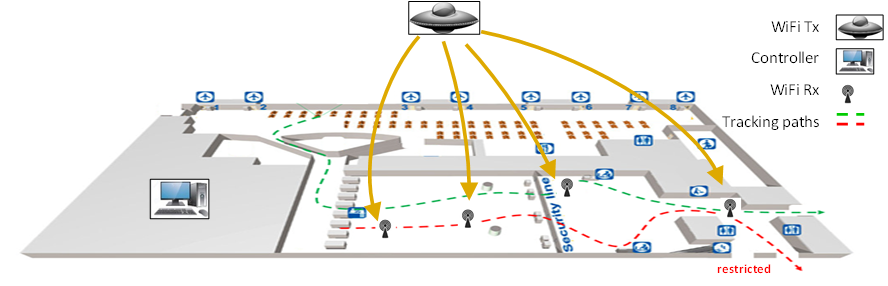
In this work, we propose Time-Reversal Indoor Tracking System (TRITS), which meets all the key demands of RTLS at the same time. A WiFi transmitter (Tx) broadcasts beacon signal to all receivers (Rx), and each Rx updates its coordinate and feeds back to controller. Then, Controller can track each Rx simultaneously. TRITS utilizes the TR resonating effect to estimate the moving distance of the object and utilizes gyroscope and accelerator to estimate direction. The information of floorplan is also exploited to correct estimation errors like landmarks. Since only local information is used and no pre-mapping is needed, TRITS is robust to environmental dynamics.
See Demo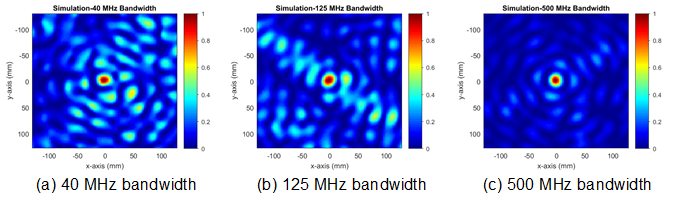
Wireless Events Detection
In the wireless transmission, when an EM signal travels over the air in a rich-scattering indoor environment, it encounters reflectors and scatters that alter and attenuate signals differently. Consequently, the received signal at the receiving antenna is a combination of multiple altered copies of the same transmitted signal coming from different paths and suffering different delays. This phenomenon is well known as multipath propagation. In order to detect an indoor event, wireless sensors should be capable of tracking the targets against all other interferences. Previous indoor wireless monitoring systems can be categorized into two classes. The first class ignores the multipath effect and only uses a single-valued CSI feature like RSSIs for detection, which leads to the degradation of accuracy to some extent. On the other hand, the second class tries to separate different components in a multipath channel, by means of UWB transmission or specially-designed frequency modulated signals.
As opposed to them, we propose a novel wireless monitoring system that monitors and detects different indoor events by utilizing time-reversal (TR) technique. As demonstrated in Figure 1, each dot in the CSI logical space represents an indoor event or location, which is uniquely determined by the multipath profile. By mapping each event into the TR space through TR operations, the similarity between two indoor events or indoor locations is quantified by the strength of TR resonances. The higher the TRRS is, the more similar two points are in the TR space. Similar multipath profiles defined by a threshold on TRRS will be treated as representing the same indoor event in the proposed system. The proposed system enables low-complexity devices with the single antenna, operating in the ISM band to detect indoor events even through the walls with a high accuracy. See Demo
Radio Human Biometrics
Nowadays, the capability of performing reliable human identification and recognition has become a crucial requirement in many applications, such as forensics, airport custom check, and bank securities. Current state-of-the-art techniques for human identification rely on the discriminative physiological and behavioral characteristics of human, known as biometrics. The well-known biometrics for human recognition include fingerprint, face, iris, and voice. Since biometrics are inherent and distinctive to an individual, biometric traits are widely used in surveillance systems for human identification. However, all of them require special devices that capture human biometric traits in an extremely line-of-sight (LOS) environment, i.e., the subject should make contact with the devices.
According to the literature, the wireless propagation around the human body highly depends on the physical characteristic (e.g., height and mass), the total body water volume, the skin condition and other biological tissues. The human-affected wireless signal under attenuations and alterations, containing the identity information, is defined as human radio biometrics . Since the probability of two individuals to have exactly the same physical and biological characteristics is extremely small, the multipath profiles after human interferences are therefore different among different persons. Consequently, human radio biometrics, which record how the wireless signal interacts with a human body, are altered accordingly to individuals' biological and physical characteristics and can be viewed as unique among different individuals.
In this work, a novel concept of radio biometrics is proposed, and accurate human identifications and verifications can be implemented with commercial WiFi devices in a through-the-wall setting by time-reversal (TR) technique. To the best of our knowledge, this is the first effort to show and verify the existence of human radio biometrics, which can be found embedding in the wireless channel state information (CSI). Moreover, we propose a human recognition system that extracts the unique radio biometrics as features from the CSI for differentiating between people through the wall. We define the term radio shot as the procedure to take and record human radio biometrics via WiFi signals. We build the first prototype that implements the TR human identification system using off-the-shelf WiFi chipsets, and test in an indoor office environment during normal working hours with an identification rate as 98.78% in identifying about a dozen of individuals.
See DemoWireless Vital Signs Detection
Breathing rate is an important vital indicator for the health status and predictor of medical conditions. Breathing monitoring is the key technology in the future medical care system. Nevertheless, most conventional breathing monitoring methods are invasive in that they require physical contact with human bodies.
Contact-free breathing monitoring schemes are developed to overcome the drawbacks of conventional schemes for in-home breathing monitoring. Among them, schemes driven by radio frequency (RF) techniques are the most promising candidates due to their abilities to sense breathing in a highly complicated indoor environment by leveraging the propagation of electromagnetic (EM) waves. Among them, schemes exploiting the channel state information (CSI), a fine-grained information that portraits the EM wave propagation, are gaining popularity due the higher resolution of CSI as well as its availability on off-the-shelf WiFi devices.
In this work, we introduce TR-BREATH, a contact-free breathing monitoring system leveraging time-reversal (TR) technique that detects and monitors multi-person breathing utilizing the CSIs obtained from off-the-shelf WiFi devices. It can work in both line-of-sight (LOS) and non-line-of-sight (NLOS) scenarios within a short period of measurement. By calculating the time-reversal resonating strength (TRRS), TR-BREATH could capture the minor but periodic variations in CSIs caused by breathing. The TRRS is further analyzed by Root-MUSIC to produce breathing rate candidates. Then, key statistics are derived based on these candidates to facilitate breathing detection. If breathing is detected, TR-BREATH estimates the multi-person breathing rates via affinity propagation, likelihood assignment, and cluster merging. Based on the cluster likelihoods, TR-BREATH could formulate an estimation on the number of people. Also, TR-BREATH makes full use of the sequence numbers in WiFi packets to enhance its robustness against packet loss which is common in areas with densely deployed WiFi devices.
We conduct extensive experiments in typical indoor environment, ranging from rooms in an office building and on campus.
See Demo
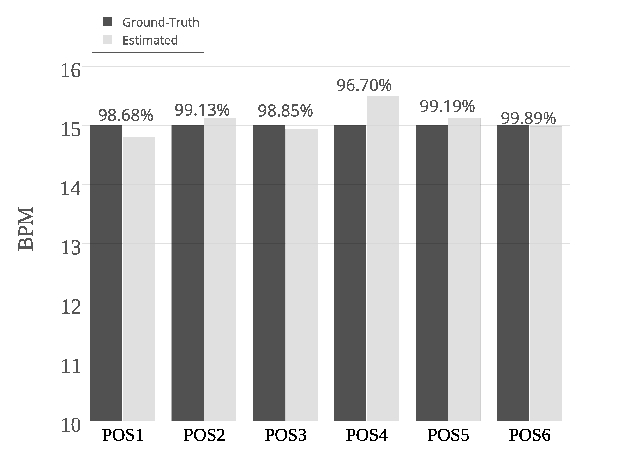
Device-free Wireless Monitoring
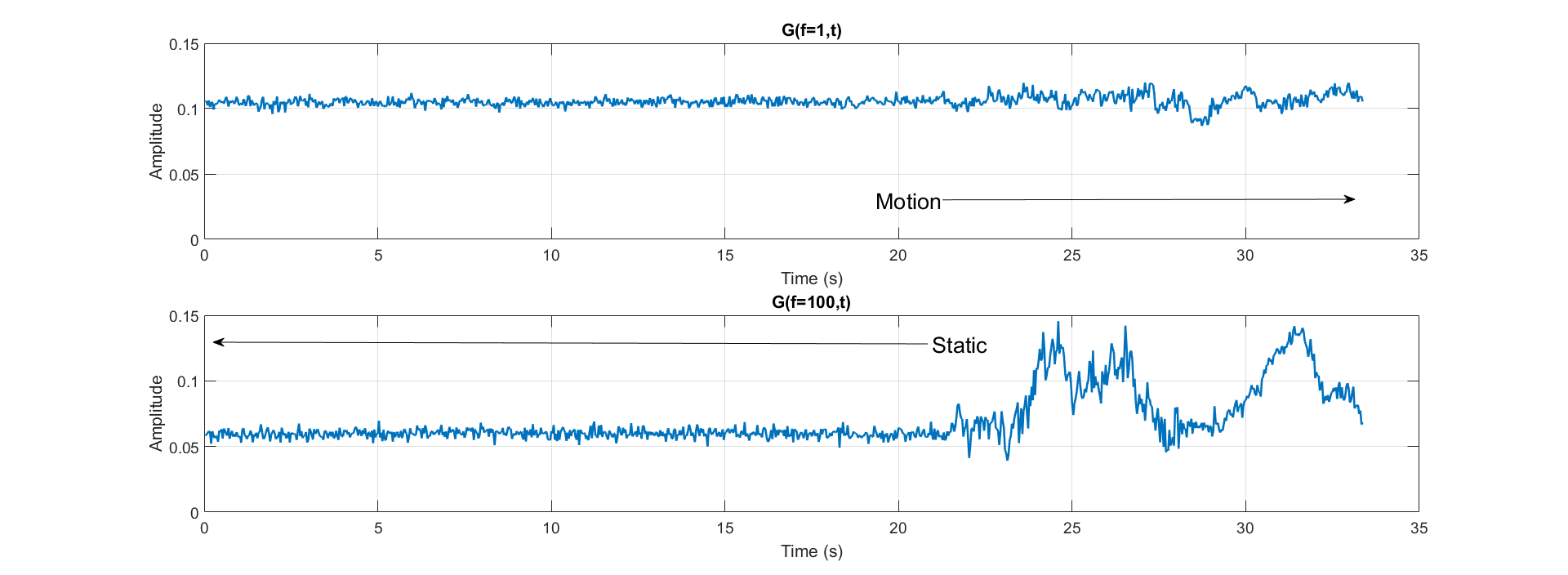
Intrusion detection plays a critical role in security of people’s possessions. Approaches such as video-based, infrared-based, RFID, UWB, etc. can provide satisfying detection accuracy. However, they all require specialized hardware deployment and strict using conditions which hinder their wide deployment. Beyond communication, WiFi can also act as generalized sensor networks and there are several research groups working on motion detection via WiFi due to its advantages in deployment flexibility, coverage, and cost efficiency. In this work, we exploit the statistical behaviors of the channel state information (CSI) measured from WiFi devices and formulate the motion detection problem to a hypothesis testing problem. Based on the proposed statistical framework, a robust, calibration-free, and low-complexity intrusion detector is constructed.
Wireless Power Transfer
In the era of Internet of Things (IoT), it is anticipated that low-power wireless devices, e.g., home appliances, security sensors, smart meters, are widely deployed as fundamental building blocks to support numerous wireless data applications. However, wireless devices are often constrained by the limited battery capacity due to massive wireless data services. In recent years, energy harvesting techniques, by which wireless devices can harvest and store energy by using rechargeable batteries, have emerged as an effective way for avoiding the cost and the challenge of battery replacement. The rapid proliferation of energy harvesting communications has urged the development of wireless power transfer (WPT) using radio frequency (RF) signals, the adoption of which as a medium is capable of wirelessly charging nodes over a long distance (up to several meters).
A major concern for designing the RF signal-based power transfer systems is the low power transfer efficiency due to the severe radio wave propagation loss, including multipath, shadowing and large-scale path loss over distance.
In this research, we propose a new concept of power transfer waveform design from the time-reversal system, called power waveforming (PW), that utilizes all available multipaths as virtual antennas to recollect all power that can be possibly harvested for power transfer. We conduct comprehensive study on the use/impact of multipaths on the wireless power transfer. An optimal energy waveform is proposed for the PW power transfer system to maximize the received power. It is discovered that a near optimal and simple PW is the single-tone waveform which only concentrates its waveform power on the principle frequency component with the highest amplitude. We also solve the problem of joint waveforming and beamforming and jointly optimize the reference signal (power signal) and the waveforms to maximize the energy delivery efficiency gain. It is shown by simulation results that compared to conventional WPT using beamforming only, the proposed approach as a 20-30dB improvement in energy delivery efficiency.
A Single-antenna Massive MIMO Solution
In a typical indoor environment, the reflection, diffraction, and scattering in the wireless medium due to the various obstacles and reflectors—such as walls, windows, and furniture—often create a large number of multipath components. As new spectrum and larger bandwidth become available, more rich-scattering multipaths can be revealed.
Different from the way conventional techniques exploit the multipath propagation environment, the TR technique can take advantage of the multipath propagation without the need for deploying complicated receivers or a large number of antennas if sufficiently large bandwidth can be used.
As shown in Figure 1(a) and 1(b), there are two ways to realize the massive MIMO effect. One is to use a large number of real antennas to straightforwardly build a massive antenna system. And the other is to leverage TR that inherently treats the multipaths in the environment as virtual antennas. Both can achieve the spatial-temporal resonance at a particular space and time that we now commonly term as the massive MIMO effect. Basically, it is a small focusing ball of energy that takes place due to the very high degree of freedom.
Therefore, by exploiting a large number of virtual antennas, a single-antenna TR system can achieve superior focusing effect in both time and spatial domains, resulting in similar promising performance as massive MIMO systems. In addition, the implementation complexity of a TR system is much lower since it utilizes the environment as a virtual antenna array and a computing resource.
MAC for 5G and beyond
The Internet of Things concept hopes to connect all the everyday devices and appliance and to provide numerous services to the users. This vision relies heavily on the ability that the communication system accommodates and coordinates the massive number of users in the system simultaneously. Thus, it is highly desirable that the coming 5G system to accommodate massive number of users simultaneously. The massive number of users, however, poses a limit on system performance due to increasing interuser interference (IUI). The 5G system therefore has to make a judicial selection of transmitting users in order to maintain the transmission quality of the users.
In SIG lab, we design novel medium access control (MAC) layer algorithms for the 5G systems. We focus on fast scheduler algorithms to coordinate the massive number of users in the upcoming 5G system. The scheduler optimization problem is transformed and formulated as a mixed integer quadratically constrained quadratic program (MIQCQP), which solves the optimization much faster than existing scheduler designs. The fast algorithm principle is also investigated for user selection and rate control to provide satisfactory services to the massive number of users.
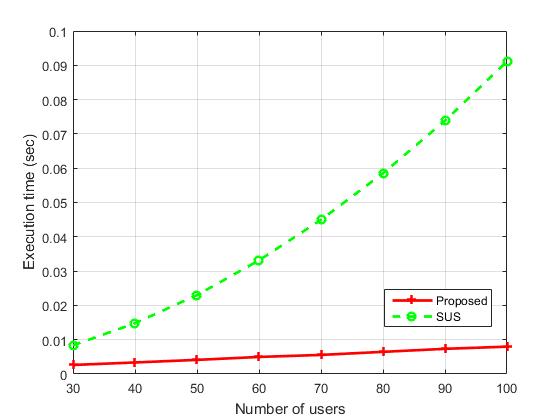